Translate this page into:
Role of antioxidants in enhancing radioprotection: Insights from diagnostic radiology and radiotherapy studies
*Corresponding author: S. Abhijith, Department of Medical Radiology and Diagnostic Imaging, School of Allied Health Sciences, REVA University, Bangalore, India. abhijithshirlal1999@gmail.com
-
Received: ,
Accepted: ,
How to cite this article: Abhijith S, Aswathi P. Role of antioxidants in enhancing radioprotection: Insights from diagnostic radiology and radiotherapy studies. Karnataka Med J. doi: 10.25259/KMJ_22_2024
Abstract
Introduction:
With recent advancements, the usage of ionising radiation in diagnostic and therapeutic radiology is becoming increasingly important. Since the smallest dose of ionising radiation can also cause biological effects, most commonly indirect effects, minimising this is the biggest challenge. The current review discussed the efficacy of antioxidants on neutralising free radicals formed as a result of radiation to minimise its effects on DNA.
Aim:
The current review aimed to evaluate the efficacy of the antioxidants in reducing the biological effects caused by ionising radiation in diagnostic and therapeutic radiology.
Materials and Methodology:
The review included the articles published in the Scopus, PubMed and Web of Science databases were used. The studies reported the effectiveness of the antioxidant administration in reducing the effects of ionising radiation effects in diagnostic and therapeutic radiology.
Results:
The evaluation methods used in this review were biochemical evaluation, histopathological evaluation, gene expression, etc. Administration of antioxidants prior to irradiation was effective in reducing biological effects. Melatonin was found to be the most commonly used antioxidant in multiple studies.
Conclusion:
Administering antioxidants before irradiation effectively reduces the biological effects. Further human studies are necessary to confirm the efficacy of the same.
Keywords
Radioprotectors
Biological effects of radiation
Biodosimetry
Antioxidants
Radiation injury
INTRODUCTION
In the past few decades, there has been a significant increase in the use of ionising radiation such as X-rays or gamma rays in both diagnostic and therapeutic radiology. The use of radiological investigation techniques, such as computed tomography or nuclear medicine imaging, has also been increasing significantly.[1-5] Numerous advancements aim to reduce radiation exposure.
Even low doses of radiation can cause stochastic effects, such as cancer or mutant DNA, since there is no threshold for radiation damage.[6] Numerous studies have found that exposure to radiation increases the risk of cancer, especially among youngsters.[7,8]
Direct and indirect effects of radiation have equal potency to cause the radiation-induced damage.[9] Free radicals [Figure 1], formed as a result of ionisation, will cause an indirect effect by breaking the covalent bond in the DNA and causing double-strand breakage. It causes either cell death or mutation.[10,11]
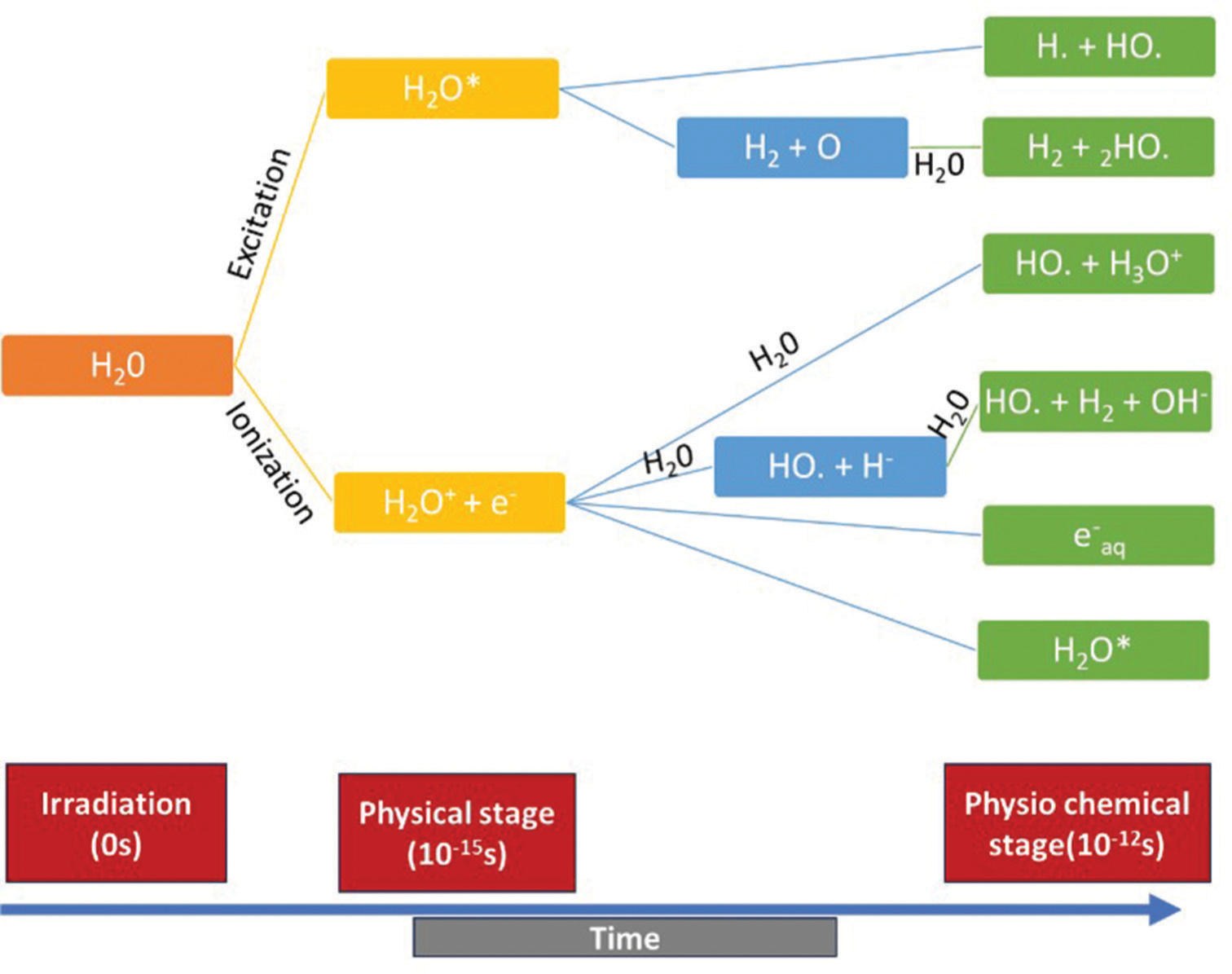
- Graphical representation of effects of ionising radiation on water molecule (indirect effect).
Antioxidants are substances that counteract free radicals by providing an electron to neutralise them [Figure 2].[12] Antioxidants such as glutathione, ubiquinol, melatonin and uric acids are produced in the body during metabolism. Some antioxidants, such as Vitamin C, Vitamin E, Selenium, Zinc and omega-3 fatty acids, must be obtained from dietary sources.[13]
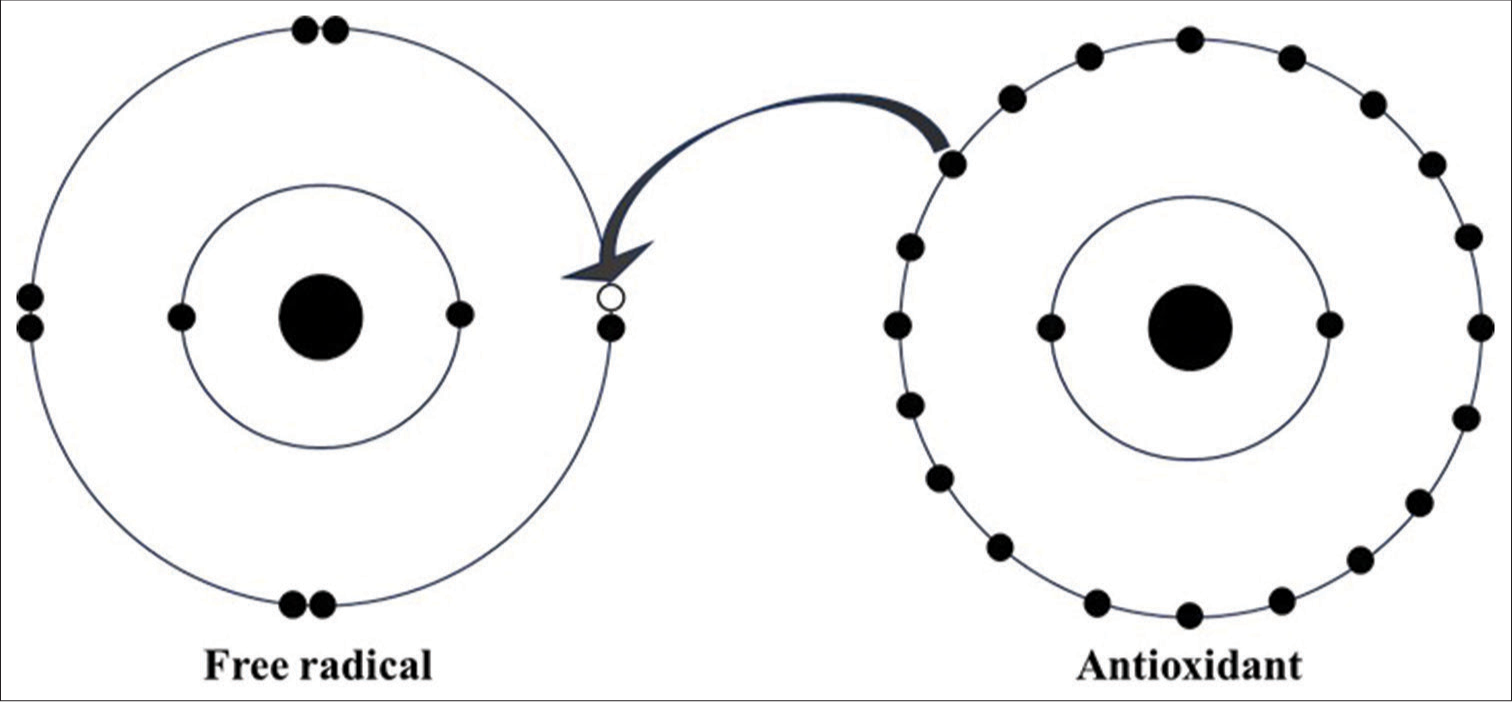
- Representation of antioxidants neutralising free radical. Black dots: Electrons, White dot: hole (vacant), Arrow: donation of electron from antioxidant to free radical.
Antioxidants could also neutralise free radicals produced due to the indirect effect of ionising radiation if it is administered before irradiation. The current article aimed to investigate antioxidants’ efficacy to protect against ionising radiation’s effects.
METHODOLOGY
Eligibility criteria
The studies reported the effect of antioxidant administration in humans or animals after irradiation with either X-rays or gamma rays in diagnostic and therapeutic radiology were included in this review.
Search strategies
This review utilised articles from the Scopus, PubMed and Web of Science databases. The search queries used were ‘antioxidant’, ‘radioprotector’, ‘X-ray’ and ‘Gamma ray,’ ‘Diagnostic radiology/radiography’ and ‘radiation therapy’. After carefully examining the titles and abstracts, the articles were selected for the final review.
Screening criteria
The study included articles that investigated the effectiveness of antioxidants in reducing DNA damage caused by radiation, either before or after the radiological procedure. A screening process was conducted by reading the titles and abstracts to ensure that the articles met the inclusion criteria. The final selection of articles was made by reading the full-length articles.
RESULTS
Study selection
A total of 1003 articles were obtained using the phrases mentioned above. These articles were carefully analysed for inclusion criteria by reading their titles and abstracts. Further, the full-length articles were read by two reviewers to determine the total number of articles that qualify for the final review, which turned out to be 19 articles [Figure 3]. These selected articles contained data on antioxidants’ efficacy in reducing radiation’s effects in diagnostic and therapeutic radiology. Articles that were not available in English and those without full text were excluded from the study.
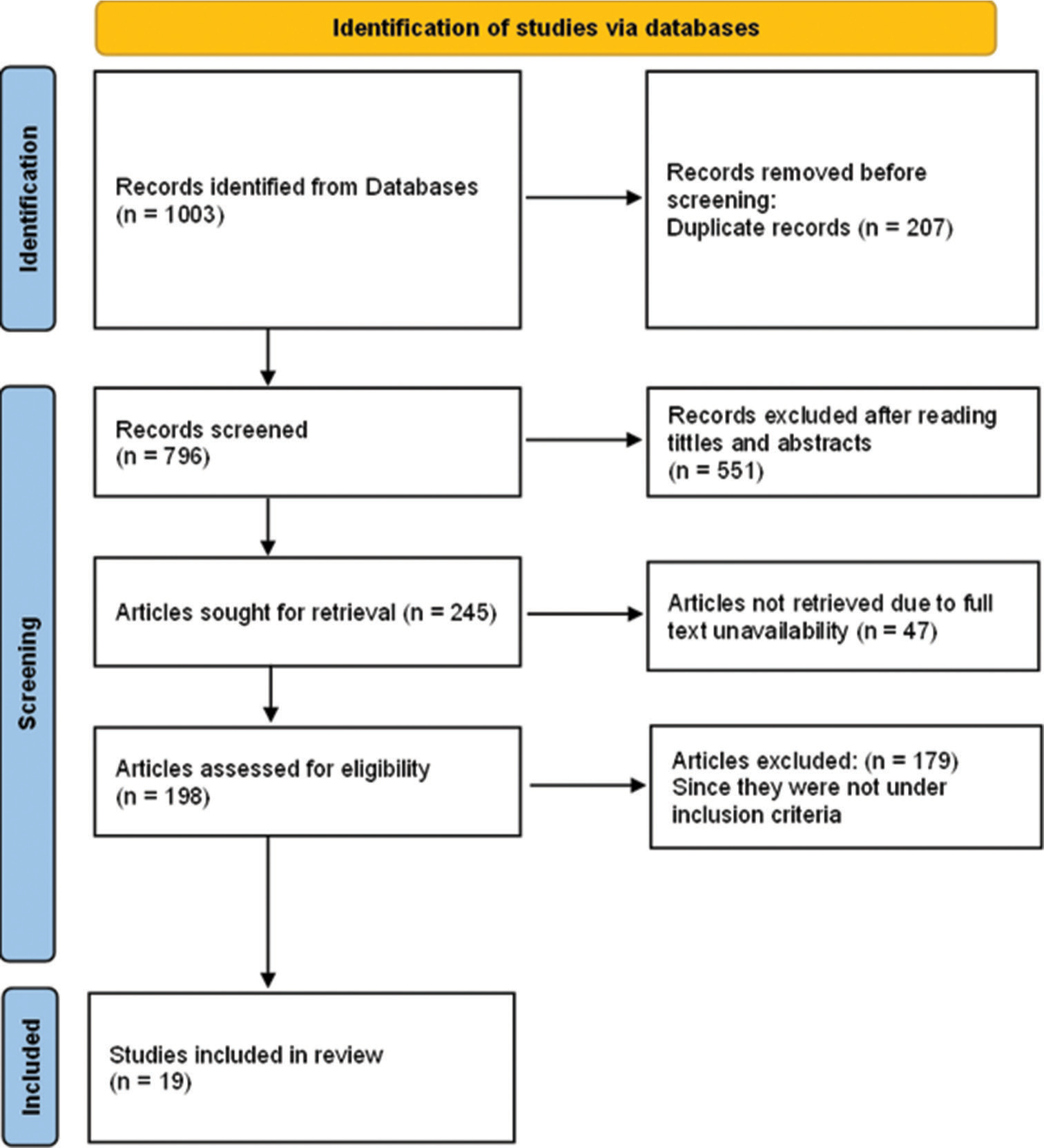
- PRISMA chart for the articles included in this review. PRISMA: Preferred Reporting Items for Systematic Reviews and Meta-Analyses.
Study characteristics
The current review included 19 original articles [14-32] conducted in Iran, Germany, Turkey, India, China, Japa, Romania and Russia. All studies were performed between the years 2012 and 2023.
ANTIOXIDANTS
Antioxidants are the molecules that readily donate electrons to free radicals to neutralise them, which otherwise would result in oxidative stress.[13] The antioxidant administration before and/or after irradiation with ionising radiation such as X-ray or gamma rays to prevent DNA damage due to oxidative stress is investigated in this review. The antioxidants in which the studies included in this review are as follows: Melatonin, Vitamin C, Vitamin E, carotenoids, genistein, N-acetyl L-cysteine, selenium and metformin.
ANTIOXIDANTS AS RADIOPROTECTORS
Protection against gamma radiation
Biochemical evaluation
Biochemical evaluation revealed that melatonin (melatonin 100 mg/kg) administration before irradiation with gamma radiation (2 Gy, 8 Gy and 30 Gy) significantly decreased malondialdehyde (MDA), lactate dehydrogenase (LDH), troponin, gamma glutamyl transferase (GGT), serum glutamic-oxaloacetic transaminase (SGOT), serum glutamicpyruvic transaminase (SGPT) and alkaline phosphatase (ALP) and significantly increased superoxide dismutase (SOD) and catalase (CAT) activity.[16,17,19,23]
Histological evaluation
In rats’ intestinal histological evaluation after irradiation with 10 Gy gamma radiation, melatonin (100 mg/kg) was effective in reducing the mucositis signs, infiltration of inflammatory cells, necrosis, damage to Brunner’s glands and reduction in goblet cells. Metformin, as compared to melatonin, was less effective in radioprotective properties.[15,31] Melatonin was also able to protect the villus height and number of villi in the jejunum and ileum of rats after irradiation (8 Gy).[14] A testicular evaluation of the testicular tissue showed melatonin was effective in reducing atrophy of seminiferous tubules, Leydig cell hyperplasia, basal lamina thickening, spermatogonia number reduction and Johnsen score reduction after irradiation in mice. Furthermore, melatonin in combination with metformin, was also effective in the reduction of all those parameters.[16,26] Another study for radioprotective effects of melatonin in rats showed a reduction in proliferative lesions of glomeruli and wall thickness of Bowman’s capsule in the kidney, a reduction in radiation-induced thickness of muscle fibre or muscle fibrosis in the heart and a reduction in hepatocyte ballooning and pyknotic nuclei formation in liver.[19] Furthermore, in the liver, melatonin (100 mg/kg) and genistein (200 mg/kg) were effective in reducing liver necrosis.[22]
Other types of evaluations
In male rats, after getting exposed to 5 Gy and 7.5 Gy gamma radiation, micronucleus (MN) frequency was increased significantly, and melatonin 100 mg/kg administration was able to reduce this effect significantly (P < 0.05). Moreover, Comet assay also supported the above result, in which radiation-induced DNA damage percentage was reduced significantly in melatonin-administered group (P < 0.05). Furthermore, gene expression of granulocyte colony-stimulating factor (G-CSF), Bcl-2-associated X protein and Bcl2 showed that melatonin was successful in regulating G-CSF and anti-apoptotic activity.[30]
Protection against X-rays
Biochemical evaluation
Administration of melatonin before X-ray exposure reduced the hydrogen peroxide level in mice. ATP was found restored in the melatonin-administered group after exposure. Melatonin reduces the MDA level post-irradiation, which otherwise increases in response to radiation in the cerebral cortex and spleen tissues. Furthermore, glutathione peroxidase (GPx) activity in liver and cerebral cortex tissue was restored to baseline in melatonin administered group post-radiation exposure.[29] Another study performed on resveratrol 5 mg/kg was administered before 7 Gy irradiation on rats, which showed a significant reduction in MDA, SOD and CAT levels.[32] Furthermore, administration of melatonin or MitoEbselen-2, GPx activity was found to be maintained after irradiation.[21]
Histopathological evaluation
TIG 3 and MRC 5 cells derived from human lungs are counted to evaluate the effects of melatonin and MitoEbselen 2 in protecting against X-rays. Melatonin increased the growth inhibition threshold dose from >2.5 Gy to >5 Gy. However, MitoEbselen 2 alone was not effective in increasing the threshold, but in combination with melatonin showed a similar result as melatonin alone.[21]
Gene expression
Administration of 100 mg melatonin 1 or 2 h X-ray irradiation showed a significant reduction in γH2AX foci in human lymphocytes without side effects.[24,27] Another study that administered 1 g of Vitamin C 30–120 min before the multiphasic abdominal CT examination showed 61% reduction in the increase in γH2AX foci in human lymphocytes.[20] A research article concluded that antioxidants which were able to reduce the γH2AX foci were as follows: NAC (43%), Vitamin C (25%), Q 10 (18%), ß-carotene (15%), selenium (14%) and Vitamin E (12%).[25] The result was also supported by another research article which studied multiple antioxidants and concluded that N-acetyl L-cysteine administration before X-ray examination was effective in reducing the γH2AX foci followed by Vitamin C, Vitamin E and ß-carotene.[28] Furthermore, preincubation of peripheral blood lymphocytes with antioxidants mixture (33 µg/mL calcium ascorbate, 0.027 IU/mL d-alpha tocopheryl succinate, 1 µg/mL carotenoids, 16.7 µg/mL N-acetylcysteine, 2 µg/mL R-alpha-lipoic acid and 6.7 ng/mL L-selenomethionin) for 15 min immediately after exposure to 10 Gy radiation showed 23% reduction in γH2AX foci. Furthermore, oral administration of the antioxidant mixture (500 mg calcium ascorbate, 400 IU d-alpha tocopheryl succinate, 15 mg natural mixed carotenoids, 250 mg N-acetylcysteine, 30 mg alpha-lipoic acid and 100 µg L-selenomethionine) 60 min prior irradiation led to 58% reduction in γH2AX foci.[18]
DISCUSSION
The present study reviewed the efficacy of antioxidants in protecting tissues from radiation injuries. Since the use ionising radiation is the most important part of the health-care system in diagnosing disease to treat it, minimising the biological effects of radiation is very important.[33] The study used various original articles which were performed to rule out the effectiveness of antioxidants in protecting DNA against radiation.
MDA was found to be increased in serum after irradiation with X or gamma radiation.[32,34,35] This is the product of free radical generation due to oxidative stress in biological tissue after ionising radiation irradiation.[36] LDH was found to increase after irradiation, which is another marker for any tissue damage like radiation injury or due to metastasis.[37,38] Elevated serum troponine is the marker for cardiotoxicity, which was found post-radiotherapy.[39] The review findings suggest that the administration of melatonin prior to irradiation will decrease MDA, LDH and troponine levels. Moreover, MDA level also decreased post-administration of resveratrol prior to irradiation. GGT, SGOT, SGPT and ALP are the markers which indicate the oxidative stress in liver cells.[40-43] Elevation in all these three markers was found to be decreased in melatonin administered group post-irradiation, according to the review findings. Radiation decreases the SOD, CAT levels and GPx activity, which are responsible for reaction against reactive oxygen species.[44,45] The review finding suggests that, the administration of melatonin or MitoEbselen-2 maintains these levels.
Irradiation with gamma rays causes various histological damages. In digestive system damages includes, mucositis signs, inflammation of cells, Brunner’s glands damage, goblet cells reduction, villi height and number reduction in jejunum and ileum.[15,31]In testis, atrophy of seminiferous tubules, Leydig cell hyperplasia, basal lamina thickening, spermatogonia number reduction and Johnsen score reduction are most commonly seen.[16,26] In renal system, proliferative lesions of glomeruli and wall thickness of Bowman’s capsule. Also thickness of muscle fibre or fibrosis in heart and hepatocyte ballooning, pyknotic nuclei formation and necrosis of liver are seen.[19,22] The results from this review showed 100 mg/kg of melatonin administration before irradiation was able to reverse all these effects and antioxidant genistein 200 mg/kg was able to reduce liver necrosis. Furthermore, X-ray irradiation resulted in vitro growth inhibition of TIG 3 and MRC 5 cells in human lungs after a dose of 2.5 Gy.[21] In vitro treatment of melatonin was able to increase the growth inhibition threshold to 5 Gy.
γH2AX assay is the most commonly used method to rule out the DNA double-strand breaks.[46] This method is the most sensitive and reliable method to rule out radiation-induced DNA damage.[47] The multiple studies included in the review suggested that the administration of antioxidants such as melatonin, Vitamin C, NAC, Q 10, ß-carotene, selenium and Vitamin E would result in decreased X-ray-induced γH2AX foci in human peripheral blood lymphocytes. Furthermore, a few other antioxidant’s mixtures also showed similar results as mentioned in the result. Furthermore, MN frequency and comet assay also are also mostly used techniques in biodosimetry. MN frequency and % comet tail formation increases with increase in ionising radiation dose.[48,49] Melatonin administration was able to reduce both of these, including regulation of G-CSF and anti-apoptotic activity, according to the results of the review.
More number of studies on humans are needed for a stronger conclusion of the results. Since the majority of the studies are on rats, that was the major limitation of the study. Furthermore, comparison between antioxidants on a large scale is needed to find out which antioxidants can be used.
CONCLUSION
Administration of antioxidants prior to irradiation with X-rays or gamma rays would result in decreased biological damage. Multiple antioxidants were included in this review, out of which melatonin was the most used antioxidant. It was also effective in reducing all types of radiation damage.
Authors’ contributions
The guarantor of the integrity of the entire study was done by AS Study concepts and Design were carried out by both AS and AP. Literature research and Manuscript Preparation were done by Abhijith S. Manuscript editing and critical revision were done by AP. Both authors read and approved the final manuscript
Ethical approval
The Institutional Review Board approval is not required.
Declaration of patient consent
Patient’s consent not required as there are no patients in this study.
Conflicts of interest
There are no conflicts of interest.
Use of artificial intelligence (AI)-assisted technology for manuscript preparation
The authors confirm that there was no use of artificial intelligence (AI)-assisted technology for assisting in the writing or editing of the manuscript and no images were manipulated using AI.
Financial support and sponsorship
Nil.
References
- Computed tomography--an increasing source of radiation exposure. N Engl J Med. 2007;357:2277-84.
- [CrossRef] [Google Scholar]
- Coronary artery calcification screening: Estimated radiation dose and cancer risk. Arch Intern Med. 2009;169:1188-94.
- [CrossRef] [Google Scholar]
- Exposure to low-dose ionizing radiation from medical imaging procedures. N Engl J Med. 2009;361:849-57.
- [CrossRef] [Google Scholar]
- Recurrent CT, cumulative radiation exposure, and associated radiation-induced cancer risks from CT of adults. Radiology. 2009;251:175-84.
- [CrossRef] [Google Scholar]
- The future of nuclear medicine, molecular imaging, and theranostics. J Nucl Med. 2020;61:263S-72.
- [CrossRef] [Google Scholar]
- Deterministic and stochastic effects of radiation. Cancer Ther Oncol Int J. 2018;12:31-2.
- [CrossRef] [Google Scholar]
- Radiation safety: A focus on lead aprons and thyroid shields in interventional pain management. Korean J Pain. 2018;31:244-52.
- [CrossRef] [Google Scholar]
- Imaging before transfer to designated pediatric trauma centers exposes children to excess radiation. J Trauma Acute Care Surg. 2016;81:229-35.
- [CrossRef] [Google Scholar]
- Contributions of the direct and indirect effects of ionizing radiation to reproductive cell death. Radiat Res. 1992;129:228-34.
- [CrossRef] [Google Scholar]
- Ionizing radiation-induced DNA injury and damage detection in patients with breast cancer. Genet Mol Biol. 2015;38:420-32.
- [CrossRef] [Google Scholar]
- Ionizing radiation, antioxidant response and oxidative damage: Radiomodulators. Antioxidants (Basel). 2023;12:1219.
- [CrossRef] [Google Scholar]
- How to characterize an antioxidant: An update. Biochem Soc Symp. 1995;61:73-101.
- [CrossRef] [Google Scholar]
- Free radicals, antioxidants and functional foods: Impact on human health. Pharmacogn Rev. 2010;4:118-26.
- [CrossRef] [Google Scholar]
- The role of melatonin in preventing radiation-induced intestinal injury. J BUON. 2021;26:626-33.
- [Google Scholar]
- The radioprotective effect of combination of melatonin and metformin on rat duodenum damage induced by ionizing radiation: A histological study. Adv Biomed Res. 2019;8:51.
- [CrossRef] [Google Scholar]
- Biochemical and histopathological evaluation of the radioprotective effects of melatonin against gamma ray-induced skin damage. Curr Radiopharm. 2019;12:72-81.
- [CrossRef] [Google Scholar]
- Evaluation of the radioprotective effects of melatonin against ionizing radiation-induced muscle tissue injury. Curr Radiopharm. 2019;12:247-55.
- [CrossRef] [Google Scholar]
- Effect of antioxidants on X-ray-induced γ-H2AX foci in human blood lymphocytes: Preliminary observations. Radiology. 2012;264:59-67.
- [CrossRef] [Google Scholar]
- Radioprotective effects of melatonin on gamma radiation-induced tissue damage in rats. J Adv Biomed Sci. 2023;13:102-15.
- [CrossRef] [Google Scholar]
- The effect of prophylactic oral vitamin C use on DNA double-strand breaks after abdominal contrast-enhanced CT: A preliminary study. Eur J Radiol. 2019;117:69-74.
- [CrossRef] [Google Scholar]
- Melatonin and MitoEbselen-2 are radioprotective agents to mitochondria. Genes (Basel). 2022;14:45.
- [CrossRef] [Google Scholar]
- Protective effects of genistein and melatonin on mouse liver injury induced by whole-body ionising radiation. Mol Clin Oncol. 2019;10:261-6.
- [CrossRef] [Google Scholar]
- Protective effect of melatonin against radiotherapy-induced small intestinal oxidative stress: Biochemical evaluation. Medicina (Kaunas). 2019;55:308.
- [CrossRef] [Google Scholar]
- Melatonin a promising candidate for DNA Double-stranded breaks reduction in patients undergoing abdomen-pelvis computed tomography examinations. Anticancer Agents Med Chem. 2020;20:859-64.
- [CrossRef] [Google Scholar]
- Influence of different antioxidants on X-ray induced DNA double-strand breaks (DSBs) using γ-H2AX immunofluorescence microscopy in a preliminary study. PLoS One. 2015;10:e0127142.
- [CrossRef] [Google Scholar]
- Radioprotective effect of a combination of melatonin and metformin on mice spermatogenesis: A histological study. Int J Reprod Biomed. 2020;18:1073-80.
- [CrossRef] [Google Scholar]
- The radioprotective effect of melatonin against radiation-induced DNA double-strand breaks in radiology. J Cancer Res Ther. 2020;16:S59-63.
- [CrossRef] [Google Scholar]
- Effect of different antioxidants on X-ray induced DNA double-strand breaks using γ-H2AX in human blood lymphocytes. Health Phys. 2020;119:101-8.
- [CrossRef] [Google Scholar]
- Radioprotective and radiomitigative effects of melatonin in tissues with different proliferative activity. Antioxidants (Basel). 2021;10:1885.
- [CrossRef] [Google Scholar]
- Role of melatonin mediated G-CSF induction in hematopoietic system of gamma-irradiated mice. Life Sci. 2022;289:120190.
- [CrossRef] [Google Scholar]
- Protection from radiation-induced damage in rat's ileum and colon by combined regimens of melatonin and metformin: A histopathological study. Antiinflamm Antiallergy Agents Med Chem. 2020;19:180-9.
- [CrossRef] [Google Scholar]
- Protective effects of resveratrol against X-ray irradiation by regulating antioxidant defense system. Radioprotection. 2018;53:293-8.
- [CrossRef] [Google Scholar]
- Clinical applications of ionizing radiation In: Radiation in medicine: A need for regulatory reform. United States: National Academies Press; 1996. Available from: https://www.ncbi.nlm.nih.gov/books/nbk232715 [Last accessed on 2024 Jan 29]
- [Google Scholar]
- Indicators of oxidative stress after ionizing and/or non-ionizing radiation: Superoxid dismutase and malondialdehyde. J Photochem Photobiol B. 2012;117:111-4.
- [CrossRef] [Google Scholar]
- Pre and post radiotherapy serum oxidant/antioxidant status in breast cancer patients: Impact of age, BMI and clinical stage of the disease. Rep Pract Oncol Radiother. 2016;21:141-8.
- [CrossRef] [Google Scholar]
- The biological significance of malondialdehyde determination in the assessment of tissue oxidative stress. Life Sci. 1991;48:301-9.
- [CrossRef] [Google Scholar]
- The effect of x-irradiation on the lactate dehydrogenase level in plasma and in various organs of mice. Radiat Res. 1968;34:411-20.
- [CrossRef] [Google Scholar]
- Serum lactate dehydrogenase (LDH) activity following exposures to cadmium and/or 60Co gamma irradiation. J Environ Sci Health A Environ Sci Eng. 1983;18:483-92.
- [CrossRef] [Google Scholar]
- Detection of radiation induced cardiotoxicity: Role of echocardiography and biomarkers. Rep Pract Oncol Radiother. 2020;25:327-30.
- [CrossRef] [Google Scholar]
- Radiation-induced upregulation of gamma-glutamyltransferase in colon carcinoma cells is mediated through the Ras signal transduction pathway. Biochim Biophys Acta. 2006;1760:151-7.
- [CrossRef] [Google Scholar]
- Serum glutamic-oxaloacetic transaminase and glutamic-pyruvic transaminase activity in premature and full-term asphyxiated newborns. Biol Neonate. 1985;47:61-9.
- [CrossRef] [Google Scholar]
- Alkaline phosphatase In: StatPearls. Treasure Island, FL: StatPearls Publishing; 2024. Available from: https://www.ncbi.nlm.nih.gov/books/nbk459201 [Last accessed on 2024 Jan 30]
- [Google Scholar]
- Oxidative stress, DNA damage, and antioxidant enzyme activity induced by hexavalent chromium in Sprague-Dawley rats. Environ Toxicol. 2009;24:66-73.
- [CrossRef] [Google Scholar]
- Therapeutic potentials of superoxide dismutase. Int J Health Sci (Qassim). 2018;12:88-93.
- [Google Scholar]
- Radiation induced DNA double-strand breaks in radiology. Rofo. 2015;187:872-8.
- [CrossRef] [Google Scholar]
- The micronucleus assay as a biological dosimeter of in vivo ionising radiation exposure. Mutagenesis. 2011;26:11-7.
- [CrossRef] [Google Scholar]